Good Measures Methodology
Climate change
Changes in the planet’s surface and ocean temperature are a natural part of its lifecycle. These fluctuations bring changing weather and climate patterns, the emergence of new species of plants and animals and the extinction of existing species that fail to adapt to the novel environment.
However, since the 1850s onwards, heavily influenced by the advent of the man-made industrial revolution, average global temperatures have increased by 1.1oC (Figure 1, (1)).
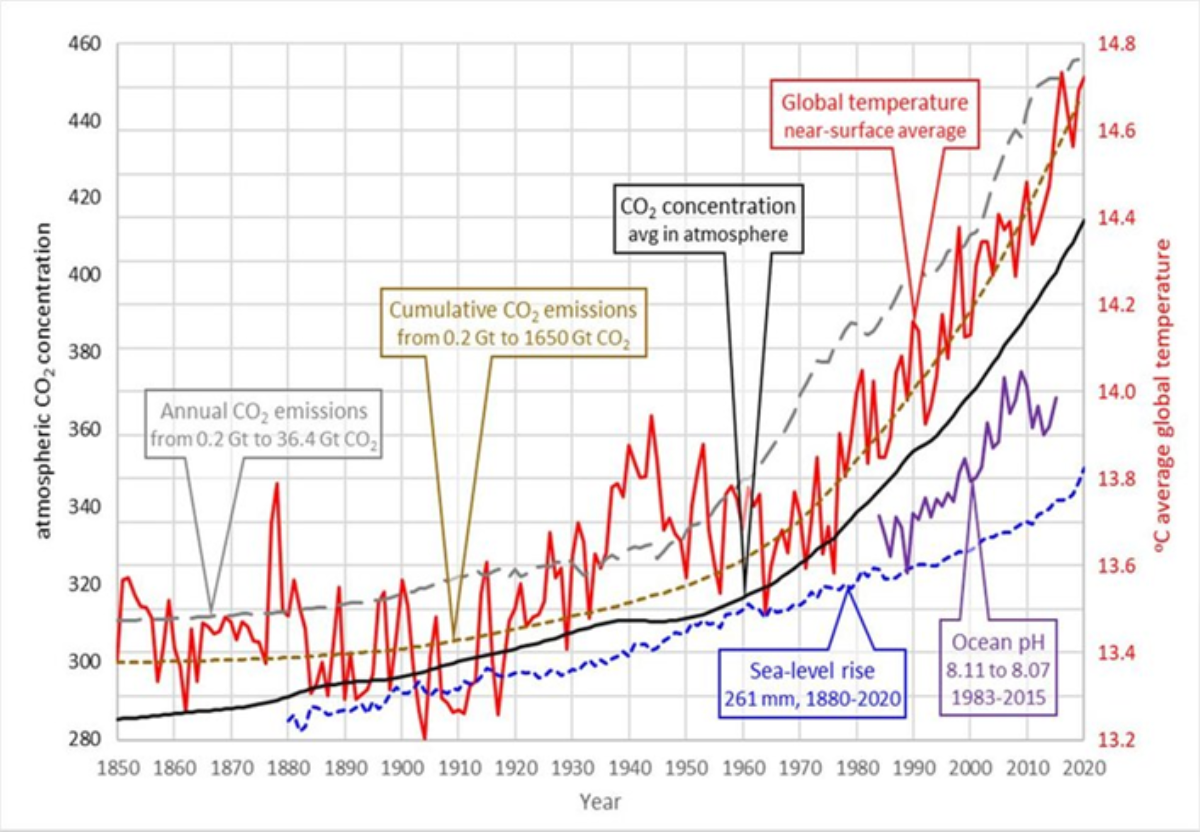
The process of increasing global temperatures is known as “Global Warming” and is driven by the “Greenhouse effect. Global warming occurs due to the heating of the gases in our atmosphere by the rays of the sun. The delicate balance of this process is essential for the existence of life on Earth.
Greenhouse Gas Emissions
Greenhouse Gas (GHG) Emissions, sometimes known as “Carbon emissions” refer to the release of GHGs, primarily carbon dioxide (CO2), into the Earth's atmosphere. These emissions are a major driver of global warming and climate change. GHG emissions have two primary sources: natural processes and human activities. Natural processes, such as volcanic eruptions and the respiration of plants and animals, release GHGs into the atmosphere. However, human activities have dramatically increased the rate of GHG emissions, particularly from the burning of fossil fuels, deforestation, and various industrial processes (Figure 1).
Fossil fuels, including coal, oil, and natural gas, release large amounts of GHGs when utilised for energy production, transportation, and heating. This is a significant driver of global warming, as the excess CO2 accumulates in the atmosphere and traps heat. Additional greenhouse gases include methane (CH4) and nitrous oxide (N2O), released from various sources like agriculture and industrial processes.
The projected consequences of unchecked increased GHG emissions are severe, leading to a range of environmental and societal challenges, including increasing global temperatures, rising sea levels, more frequent and intense extreme weather events, and disruptions to ecosystems.
Digital Advertising and Climate Change
The Information and Communication Technology (ICT) has revolutionised the way global industries conduct their day-to-day activities, however, this comes with an often-unseen cost to the environment. According to a recent analysis in 2020, the ICT sector was responsible for around 4% of global electricity consumption from the usage of servers, networks and digital devices which amounted to 1.4% of global GHG emissions (3). However, depending upon the study and estimation scope and methodology the carbon footprint of the ICT industry may be as high as 2.8%(4).
Since the advent of the internet, the digital advertising industry has gained prominence and grown substantially in size. In 2022, revenue from the digital advertising was an estimated $917 billion and is projected to grow to $1171 billion US dollars by 2028 (5).
While digital advertising eliminates some of the physical aspects and associated GHG emissions, it introduces a host of new digital processes and contributes to the overall global carbon footprint of the ICT industry.
One of the primary contributors to the release of GHG emissions from the digital advertising industry is the operation of data centres, for hosting and delivering digital content. These data centres require substantial amounts of energy for cooling, maintenance, and the functioning of servers and networking equipment.
Furthermore, the devices used to display digital ads, such as computers, laptops, smartphones, connected TV and tablets, also use energy. The energy usage of end-user devices is a significant part of the carbon footprint associated with digital advertising.
With GHG emissions projected to increase in conjunction with digital advertising revenue, there is an urgent need to stop and consider the environmental implications. To address the issue of GHG emissions and mitigate global warming, international efforts such as the Paris Agreement, adopted in December 2015, aim to reduce emissions and transition to more sustainable and renewable sources of energy.
The Paris Climate Agreement aims to strengthen the global response to the threat of climate change by keeping the global temperature rise this century well below 2 degrees Celsius above pre-industrial levels, and to pursue efforts to limit the temperature increase to 1.5 degrees Celsius. Despite the agreement’s ambitious targets, current progress is insufficient to meet these goals. Reports indicate that global emissions are not declining at the necessary rate. The latest analysis suggests that global greenhouse gas emissions need to be cut by 43% by 2030 relative to 2019 levels to meet the Paris targets (Figure 2, 6).
Therefore, it is essential that digital advertising, as a $917 billion dollar industry, take action to reduce its overall environmental impact. However, before we can start to limit this impact it is essential to first understand the various processes involved and gauge.
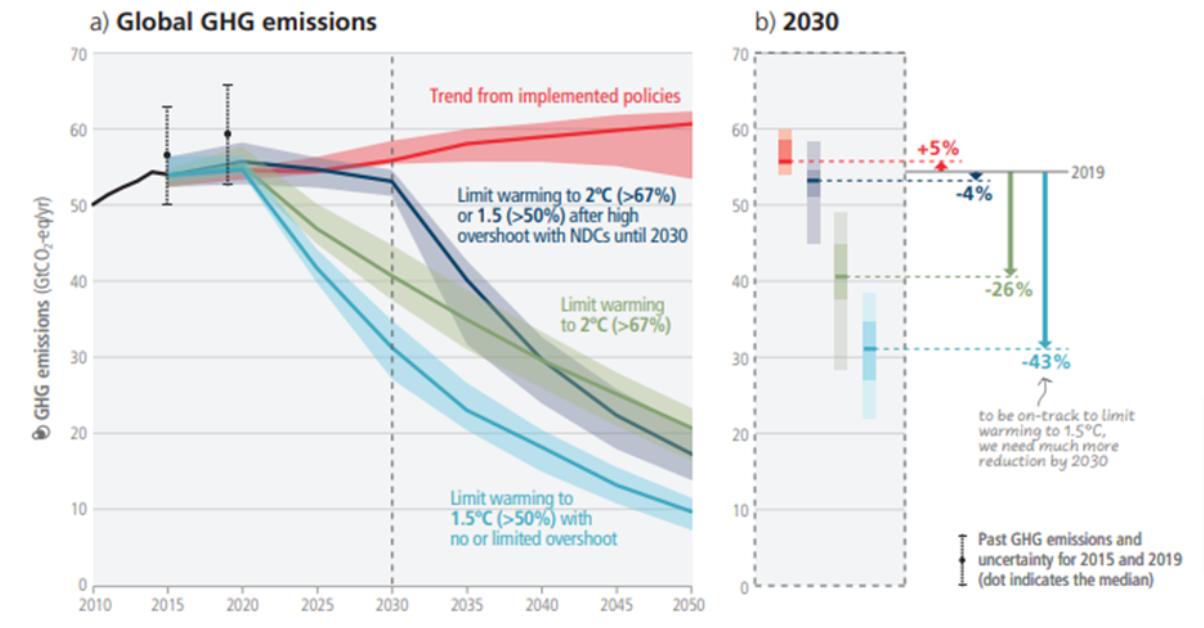
Summary
Climate change is a global problem and action is required from all major industries to reduce or reverse global temperature increases.
Digital Advertising is a huge industry with a large environmental footprint.
To reduce the environmental footprint of digital advertising and help meet global reduction goals a standardised approach to GHG estimation from the advertising industry is required.
Estimating GHG emissions from the Digital Advertising IndustryOver the past year, Good-Loop have been working with WFA and AdNetZero and other industry experts to develop a robust framework for estimating the environmental impact from digital advertising.
The voluntary framework is an omni-channel solution, for both physical and digital media, and forms the foundation from which all industry estimation standards should be based. In essence it is the blueprint for conducting life cycle assessment (LCA) from digital advertising campaigns. An LCA is defined as a systematic analysis of the environmental impact over the course of the entire lifecycle of a product material, process or other measurable activity.
The framework was created for an important reason; to standardise and improve the precision of GHG estimation models of physical and digital advertising campaigns.
Prior to the introduction of the framework, there was misalignment within the industry on the scope of the estimation, calculation inputs and even naming conventions for emissions sources. These differences were highlighted in the IAB Europe Gap Analysis published in March 2024 (Figure 3, (7)
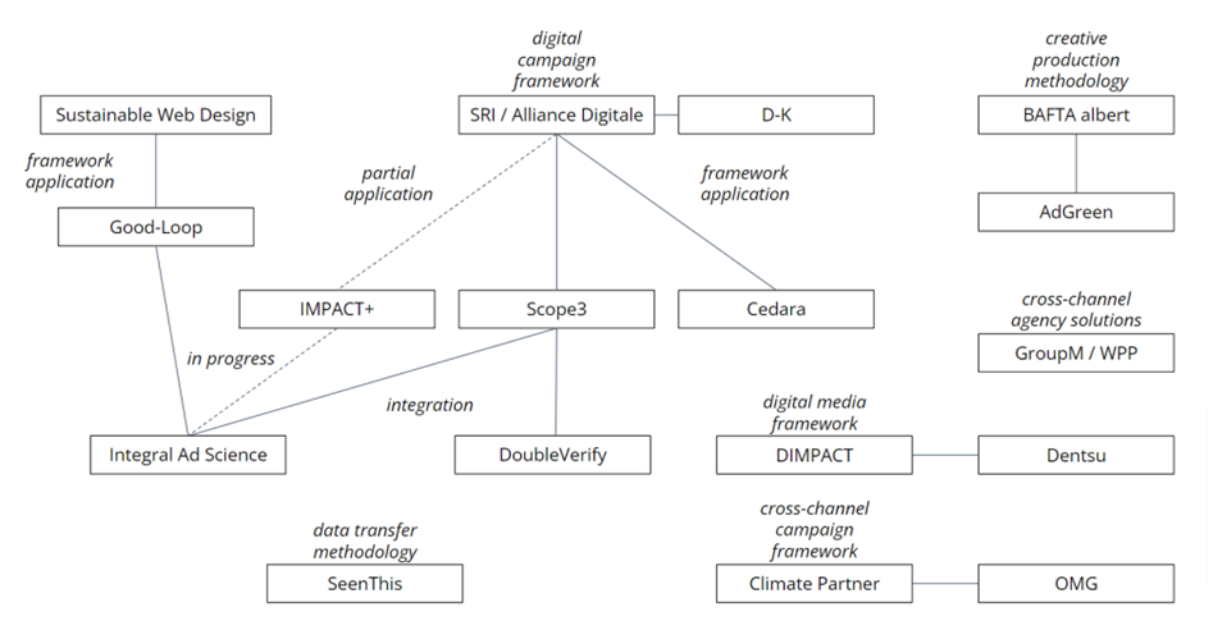
One of the main issues with the existence of several estimation framework and methodologies is that the absolute GHG number from an ad campaign varied greatly depending on the solution provider, the solution framework and calculations used. Now, industry bodies with the help of climate experts have defined the scope, and structure of the framework and have provided the equations to enable representative and consistent GHG emissions estimates.
In the interest of industry alignment, we have completely reworked not only our GHG emissions framework and methodology but also our technical infrastructure to seamlessly integrate with the sustainability framework, resulting in our industry-aligned GHG model Good Measures, which enables easy estimation and insight into the emissions from digital advertising.
But how does this work in practice?
Well, we could send you a link to our website to thousands of lines of Python code and let you figure it out for yourself, but in the interest of education, understanding and transparency. We will outline the key principles underlying our methodology and provide you with our first take on the data and assumptions we use to power our GHG model.
At this point it’s important to state that the current version of the sustainability framework is a work in progress with more releases and updates in the coming months, as such we will continue to update our methodology, equations and data sources to align with the most recent version of the framework and continue to contribute to its development.
Summary
The sustainability framework is a work in progress but lays the foundation and outlines the scope and enables standardised GHG emissions estimation from digital advertising campaigns.
It is a flexible framework in which to build GHG emissions models, however, aligning with the framework does not mean adoption of the framework in its entirety rather it means clearly stating if and how your estimation methodology differs from the framework.
Our implementation of the WFA and AdNetZero Framework v1.0 – Good Measures
At Good-Loop, we have integrated the Digital framework equations for display, search and social media ad campaigns into our GHG model, having migrated from our previous equations based on the Sustainable Web Design Methodology and the SRI framework (8,9).
Our current methodology calculates the GHG emissions from the processes involved in the Distribution and Consumption of an ad.
The full list of equations is available in the framework (10) but at its simplest level, the emissions produced during the Distribution and Consumption stages of an ad’s life cycle consist of the use-phase emissions and embodied GHG emissions from digital devices and infrastructure.
What units are used to estimate GHG emissions?
The units of estimation to estimate GHG emissionsare carbon dioxide equivalent (CO2e) and CO2e per thousand impressions (CO2e PM).
CO2e is a unit for estimating carbon footprints. It expresses the impact of GHGs in terms of the amount of CO₂ that would have the same global warming effect. This allows for the comparison and aggregation of emissions from various greenhouse gases.
CO2e PM refers to the normalised impact, in terms of CO2e, per thousand impressions from an ad campaign (10).
What are Use-Phase Emissions?
Use-phase emissions are the GHG emissions from the energy consumed by digital infrastructure such as servers, networks and user devices when they are utilised.
One example would be the GHG emissions from the energy used by a server when transmitting an ad creative to a user device, which would fall under distribution emissions.
Another example, the GHG emitted in producing the electricity used by a PC when consuming ad content, would fall under the consumption emissions category.
What are Embodied Emissions?
Embodied GHG emissions, in the context of the WFA framework, are the emissions from the manufacturing, transport and end-of-life disposal of digital devices and infrastructure.
For example, the emissions from the manufacture of a server, the transport of the server to the business or user and the emissions used from the disposal of the server once it has reached the end of its useful life.
Distribution GHG Emissions
Distribution, as defined by the digital framework, involves the processes of Ad Selection and Creative Delivery.
Ad Selection includes the processes of Real-Time Bidding (RTB), Direct Sales, Creative Selection and Placement, and Targeting.
(Note: Version 1.0 of the digital framework does not yet include equations for targeting and creative selection as such these have not been incorporated into our current calculations.)
RTB / Direct Sale Equations
Equation 1 – RTB Server Use Phase Emissions
= Impressions ∗ Number of potential active paths per impression ∗ Avails ratio ∗ (1 + Requests ratio) ∗ (1 + Responses ratio) ∗ Time of calculation per bid (h) ∗ Compute ratio allocated to bid processing (compute used by SSP/DSP incl. machine-learning) and reporting/analytics (%) * Total relevant infrastructure power incl. PUE (W) * Carbon intensity of electricity ( kgCO2e/kWh) ∗ (1 + overhead of other mutualized server resources ratio )
Variable Definitions
· Impressions = number of impressions
· Number of potential active paths per impression = number of activated paths defined as the connection between a server, SSP and DSP
· Avails ratio = the avail requests ratio sent from a publisher to an SSP
· Request Ratio = the ratio of avails to bid requests
· Response Ratio = the ratio of bid requests to bid responses
· Time of calculation per bid (h) = the time taken for each bid calculation
· Compute Ratio = ratio of physical resources dedicated to bid processing, reporting, machine learning, analytics and other such processes makes up a proportion of total compute power
· Total relevant infrastructure power incl. PUE (W)= the power of each server or other digital infrastructure incorporating the power usage effectiveness (PUE)
· Carbon Intensity (kgCO2e per kWh) = the emission factor based on server/infrastructure location.
· Overhead of other mutualized server resources ratio = additional mutualized infrastructure resources for other services and development activities.
Equation 2: RTB Server Embodied Emissions
Impressions ∗ Number of potential active paths per impression ∗ Avails ratio ∗ (1 + Requests ratio) ∗ (1 + Responses ratio) ∗ Time of calculation per bid (h) ∗ Compute ratio allocated to bid processing (compute used by SSP/DSP incl. machine-learning) and reporting/analytics (%) ∗ EF manufacturing and EOL of total relevant infrastructure (kgCO2e) / Average lifetime of equipment (s) ∗ (1 + overhead of other mutualized server resources ratio)
Variable Definitions
· Impressions = number of impressions
· Number of potential active paths per impression = number of activated paths defined as the connection between a server, SSP and DSP
· Avails ratio = the avail requests ratio sent from a publisher to an SSP
· Request Ratio = the ratio of avails to bid requests
· Response Ratio = the ratio of bid requests to bid responses
· Time of calculation per bid (h) = the time taken for each bid calculation in hours
· Compute Ratio = ratio of physical resources dedicated to bid processing, reporting, machine learning, analytics and other such processes makes up a proportion of total compute power
· EF manufacturing and EOL of total relevant infrastructure (kgCO2e) – the emissions produced from manufacturing and end- of life (EOL) from digital infrastructure.
· Average lifetime of equipment (s) = the average lifetime in seconds of digital infrastructure, for example, the average lifetime of RTB servers.
Equation 3: RTB Network Use Phase Emissions
= Impressions ∗ Number of potential active paths per impression ∗ Avails ratio ∗ (1 + Requests ratio) ∗ (1 + Responses ratio) ∗ Data transferred by request type (kB) ∗ Server-to-server networks energy efficiency according to network type and country (kWh/kB) ∗ Carbon intensity of electricity ( kgCO2e/kWh)
Variable Definitions
· Impressions = number of impressions
· Number of potential active paths per impression = number of activated paths defined as the connection between a server, SSP and DSP
· Avails ratio = the avail requests ratio sent from a publisher to an SSP
· Request Ratio = the ratio of avails to bid requests
· Response Ratio = the ratio of bid requests to bid responses
· Data transferred by request type (kB) = an estimate may be obtained from bid request or response size + overhead payload of additional assets.
· Server-to-server networks energy efficiency according to network type and country (kWh/kB) = network efficiency depending upon the location of servers.
· Carbon Intensity (kgCO2e per kWh) = the emission factor based on network infrastructure location.
Equation 4: RTB Network Embodied Emissions
= Impressions ∗ Number of potential active paths per impression ∗ Avails ratio ∗ (1 + Requests ratio) ∗ (1 + Responses ratio) ∗ Data transferred by request type (kB) ∗ EF manufacturing & EOL amortization networks according to network type and country (kgCO2e/kB)
Variable Definitions
· Impressions = number of impressions
· Number of potential active paths per impression = number of activated paths defined as the connection between a server, SSP and DSP
· Avails ratio = the avail requests ratio sent from a publisher to an SSP
· Request Ratio = the ratio of avails to bid requests
· Response Ratio = the ratio of bid requests to bid responses
· Data transferred by request type (kB) = an estimate may be obtained from bid request or response size + overhead payload of additional assets.
· EF manufacturing & EOL amortization networks according to network type and country (kgCO2e/kB) = the emissions produced from manufacturing and end- of life (EOL) from digital infrastructure, in this case mobile or fixed networks.
Creative Delivery
Creative Delivery refers to the transmission of a creative asset across networks and processing through ad servers and edge nodes up to the point of being displayed on a user device.
Equation 5: Creative Transmission Server/Edge Node Use-Phase Emissions
= Impressions ∗ total server output data per impression (kB) *
Breakdown of content delivered by ad servers vs. edge nodes (%) ∗ datacentre or edge nodes energy of efficiency including PUE (kWh/kB output) ∗ carbon intensity of electricity (kgCO2e/kWh))
Variable Definitions
· Impressions = number of impressions
· total server output data per impression (kB) = For static ad formats, a file size proxy + payload overhead of additional assets may be used. For video formats, the portion of file size loaded (incl. buffer) + payload overhead of additional assets should be used.
· Datacentre or edge nodes energy of efficiency including PUE (kWh/kB output)
· Carbon intensity of electricity (kgC02e/kWh)) = the emission factor based on data centre or edge node location.
Equation 6: Creative Transmission Server/CDN Edge Node Embodied Emissions
= Impressions ∗ Total server output data per impression (kB) *
Breakdown of content delivered by ad servers vs. edge nodes (%) ∗ EF manufacturing and EOL of total relevant infrastructure (kgCO2e) / infrastructure output bandwidth (kB/s) / average lifetime infrastructure equipment (s)
Variable Definitions
· Impressions = number of impressions
· total server output data per impression (kB)
· EF manufacturing and EOL of total relevant infrastructure (kgCO2e) = the emissions produced from manufacturing and end- of life (EOL) from digital infrastructure, in this case servers or CDN edge nodes.
· Infrastructure output bandwidth (kB/s) = output bandwidth of servers or edge nodes
· Carbon intensity of electricity (kgC02e/kWh)) = the emission factor based on data centre or edge node location.
Equation 7: Creative Transmission Network Use-Phase Emissions
= Impressions ∗ total data transferred on network per impression (kB)
∗ consumption breakdown between types of networks (%) ∗ energy efficiency according to network type and country (kWh/kB)
* energy efficiency according to network type14 and country (kWh/kB))
* consumption breakdown between countries of servers/edges nodes and & users(%) * carbon intensity of electricity (kgCO2e/kWh))
Variable Definitions
· Impressions = number of impressions
· total data transferred on network per impression (kB) = For static ad formats, a file size proxy + payload overhead of additional assets may be used. For video formats, the portion of file size loaded (incl. buffer) + payload overhead of additional assets should be used.
· Energy efficiency according to network type and country (kWh/kB) = the energy of fixed or mobile networks depending upon network location
· Carbon intensity of electricity (kgC02e/kWh) = the emission factor based on network location.
Equation 8: Creative Transmission Network Embodied Emissions
= Impressions ∗ Total data transferred on network per impression (kB) *
consumption breakdown between types of networks (%)
∗ EF manufacturing & EOL amortization networks according to network type and country (kgCO2e/kB)
Variable Definitions
· Impressions = number of impressions
· Total data transferred on network per impression (kB) = For static ad formats, a file size proxy + payload overhead of additional assets may be used. For video formats, the portion of file size loaded (incl. buffer) + payload overhead of additional assets should be used.
· EF manufacturing & EOL amortisation networks according to network type and country (kgCO2e/kB) = the emissions produced from manufacturing and end- of life (EOL) from digital infrastructure, in this case fixed or mobile networks.
Consumption
Consumption emissions are produced from viewing an ad on a user device such as a smartphone or CTV. This includes the electricity used during the viewing of an ad and the embodied emissions from the user device.
Equation 9: User Device Power Use-Phase Emissions
= Device mix by type and country (%) ∗ Time displayed on device (s) ∗ Device render power consumption (W) ∗ time conversion ratio (h/s) ∗ carbon intensity of electricity (kgCO2e/kWh))
Variable Definitions
· Device mix by type and country (%) = for the overall campaign this is the percentage of device an ad was viewed on in each country. For example, 5% of views were on PC in the UK.
· Time displayed on device (s) = the viewing time of the ad.
· Device render power consumption (W) = the power consumption value for each device type.
· Time conversion ratio (h/s) = the conversion factor from hours to seconds.
· Carbon intensity of electricity (kgCO2e/kWh)) = the emission factor based on the user device location.
Equation 10: User Device Power Embodied Emissions
= Device mix by type and country (%) ∗ Time displayed on device (s) ∗ EF manufacturing & and EOL amortization of devices (kgCO2e/unit) / total active used time over lifetime by device type (s of active use over full lifetime)
Variable Definitions
· Device mix by type and country (%) = for the overall campaign this is the percentage of device an ad was viewed on in each country. For example, 5% of views were on PC in the UK.
· Time displayed on device (s) = the viewing time of the ad.
· EF manufacturing & and EOL amortization of devices (kgCO2e/unit) = the emissions produced from manufacturing and end- of life (EOL) from digital user devicesfor example PC or smartphone.
· Total active used time over lifetime by device type (s of active use over full lifetime) = the average active use time of the device over the course total useful life of the device. For example, daily device usage of 2 hours per day over the course of 5 years.
Data Defaults and “Data Hacks”
To power the Digital Framework formula, it is essential to have quality, reliable data and we actively work with advertisers to obtain the most recent first party data relating to their GHG emissions. Ideally this would be at the media product level. However, in some cases data is unavailable and certain assumptions and reasonable estimations must be made. The framework outlines a specific set of criteria to reduce data uncertainty and improve data transparency (outlined below).
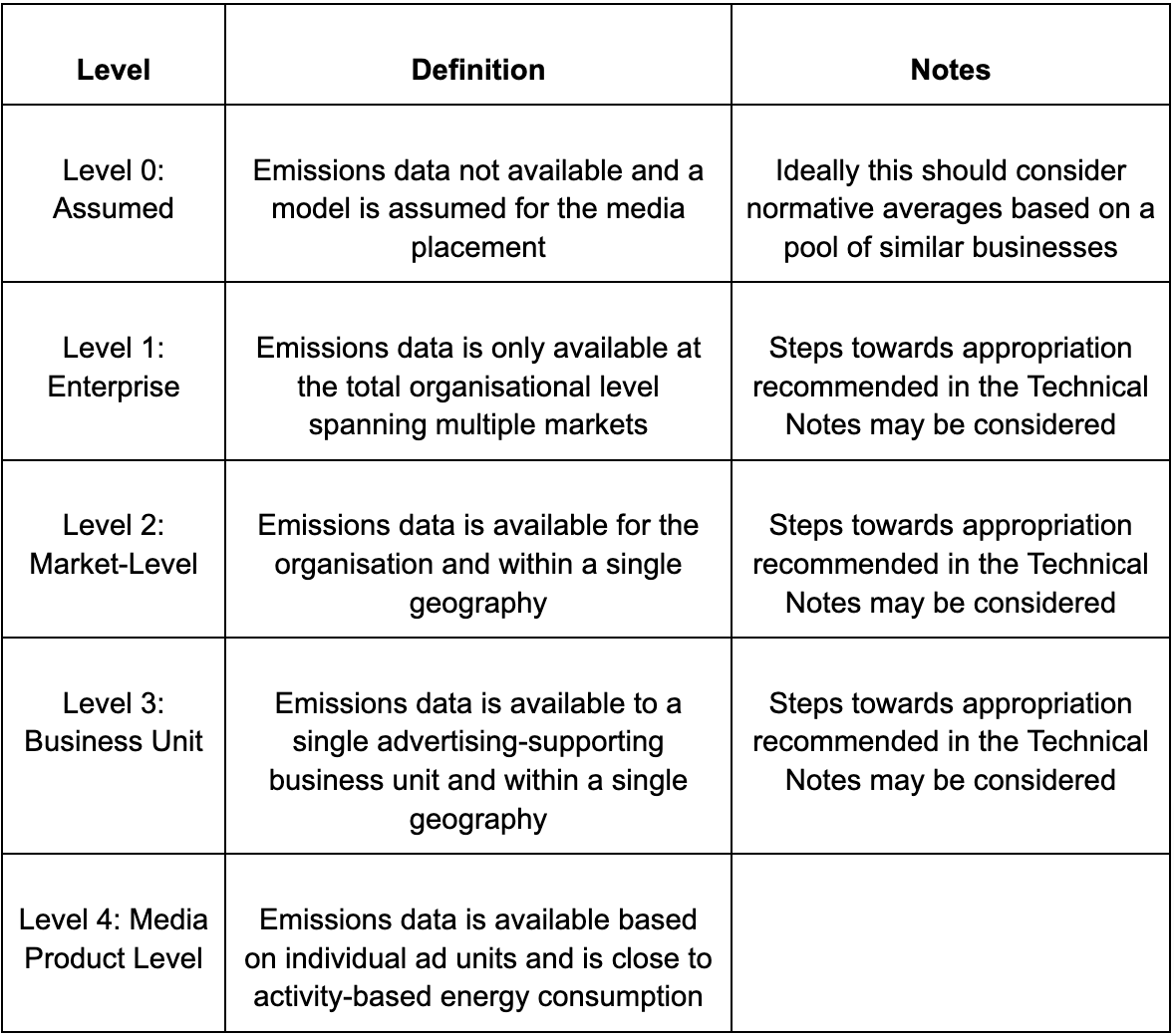
Below we have included a list of Level 0: Assumed data that we use to power our GHG model in its current iteration. We believe that values we utilise are conservative however we will continue to update this with more recent data as and when it becomes available.
The Good Measures Tag – an easy way to attain Media Product Level Data
To allow advertisers to attain the highest quality data to power the GHG model, they can utilise our Good Measures Tag at the level of a single impression.
For example, using the Good Measures Tag, we can attain location data at country and state level and device data such as device type and manufacturer. This can allow granular insights at the Media Product Level.
Emission Factors/ Carbon Intensity Values – what are these and why are they important to the Sustainability framework?
Emission factors or energy intensity factors are an important variable in the calculation of GHG emissions in the Digital framework. Emission factors are representative values that attempt to quantify a pollutant, in this case GHG emissions, with an activity associated with that pollutant, i.e. the electricity usage attributable to digital advertising (11).
Location-based emissions factors, or carbon intensities, refer to CO2e emissions produced per unit of energy consumed, which varies significantly depending on the geographical region. These factors are influenced by the local energy mix, where regions relying heavily on fossil fuels such as coal and natural gas typically exhibit higher carbon intensities compared to those that utilize a greater proportion of renewable energy sources like wind, solar, and hydroelectric power. For instance, electricity generated in areas with abundant renewable resources will have a lower emissions factor than in regions dependent on coal-fired power plants. Understanding these variations is crucial for businesses and policymakers aiming to accurately measure and reduce their carbon footprints, enabling more targeted and effective strategies for mitigating climate change.
The type of emissions factor that should be utilised depending on numerous factors and official guidelines vary in their recommendations.
The GHG Protocol, widely used for corporate GHG reporting, also provides guidance on emissions factors in LCA. It recommends using location-based emissions factors that reflect the specific electricity grid where the energy is consumed. The protocol allows for the use of yearly average factors for general reporting but encourages the use of more granular factors (monthly or hourly) when they are relevant to the analysis.
In order to utilise the emissions factors published by EMBER as these are open access, open-source methodology and have been utilised in previous industry emissions methodologies.
Frequently Asked Questions
Why is the Creation section absent from the GHG model?
The current version of the framework does not include the Creation portion of GHG emissions as some processes such as production and postproduction are out-of-scope. The creative storage equations of the framework are also not yet in use. However, when these sections are implemented, we will update our existing model.
Do you include the Corporate Emissions as part of your GHG model?
Yes, if each identified member in the value chain provides an annual enterprise level GHG report we can incorporate this into the final GHG calculation.